Biological modeling and treatment planning
Scope of this project:
- Mathematical models for TCP and NTCP calculation
- Biological imaging via PET and MRI
- Definition of biological target volumes
- Response-adaptive radiotherapy and dose painting
Radiotherapy treatment plans are based on imaging data sets, usually acquired via computer tomography (CT), positron emission tomography (PET) and/or magnetic resonance imaging (MRI). A 3D image allows for a definition of various structures, e. g. a tumour and organs at risk, and therefore provides a treatment planning system with the possibility both to prescribe a certain dose value to a particular structure as well as to calculate the results of a treatment plan, meaning the dose values actually applied to the individual structures. In general, any treatment plan maximizes the dose to the tumour homogeneously up until the maximum dose deposition allowed for the surrounding healthy tissue is reached. A potential problem arises due to inhomogeneities in radiosensitivity within the tumour volume.
In this project, we investigate the potential of non-uniform, heterogeneous dose distributions within the tumour to account for variations in radiosensitivity. This process is called dose painting and is mainly motivated by three hypotheses.
- Highly radioresistant fractions of the tumour volume are not eradicated by a safely deliverable uniform dose distribution and can be the cause for local recurrences.
- Molecular and functional imaging like positron emission tomography (PET) allows spatio-temporal mapping of the tumour volume and provides information about the local relative radioresistance.
- Modern radiotherapy planning and delivery systems are able to deliver a graded boost to the high-risk sub-volumes, potentially increasing the tumour control probability (TCP).
The functional images required to obtain information about potential high-risk structures can be acquired using combinations of CT and PET scans. Various biomarkers, each connected to particular PET tracers, have been identified to be related directly to the therapeutic response and an increased risk of recurrence. The three most commonly considered biological characteristics are glycolytic metabolism, proliferation and oxygenation. The concept of dose painting is illustrated in figures 1 and 2 for the biomarker oxygenation.
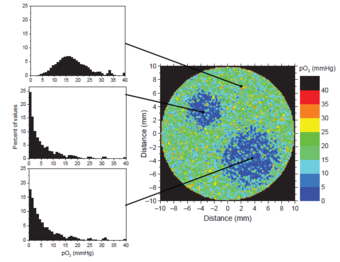
Hypoxic regions define two high-risk sub-volumes.
Hypoxic tissue (low oxygen saturation) has shown a significantly higher radioresistance than normally oxygenated tissue. Figure 1 shows the simulation of a PET scan for the oxygen partial pressure pO2 within a hypothetical tumour. The colors represent the oxygen saturation from low (blue) to normal (green) and high (red). Such information provides the possibility to define two high-risk sub-volumes, or boost-volumes, which should receive a higher dose than the rest of the tumour. A resulting dose prescription might look like the one illustrated in figure 2.
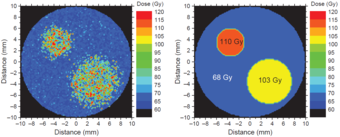
Dose painting within the tumour volume: hypoxic regions receive higher doses than the rest of the tumor.
To take into account the uncertainties of the pre-treatment imaging, the dynamics of the biological system during the treatment period and the radiation-induced biological changes of the tumour, our research also covers the concept of adaptive radiotherapy based on additional functional imaging during the treatment period. While direct monitoring of the delivered dose during treatment is not yet possible, monitoring the tumour response does indeed seem feasible via repeated PET imaging. This kind of response assessment could eventually allow for an individually adapted radiotherapy based on functional imaging during the therapeutic process. The concept of response-adaptive radiotherapy is illustrated in figure 3. In addition to the first image acquisition at baseline (time t0), on which the initial treatment plan is based, further images are taken a couple of weeks into the treatment period (time t1, t2) in order to investigate the response to the treatment and, if necessary, to adapt the treatment plan.
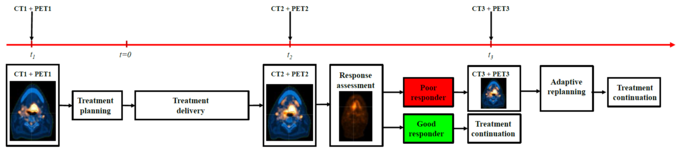
Schematic representation of a response-adaptive radiotherapy.
These techniques have the potential to provide a paradigm shift in radiation treatment planning and to substantially impact clinical outcomes. With the ability to not only consider the pre-treatment conditions of patients but also their intrinsic responsiveness to the treatment, adaptive dose painting based on PET imaged biomarkers provides an important step towards biologically guided radiation therapy. However, there is still a lot of research to do in order to make response-adaptive radiotherapy and dose painting feasible for clinical routine.
In this project, we closely collaborate with our colleagues from the Karolinska Institutet in Stockholm.